(English below)
El RNA funciona como transportador de la información desde el DNA hacia las proteínas a través de mecanismos complejos. Pero en lugar de codificar proteínas, ciertas moléculas de RNA regulan su expresión o cumplen otras funciones no codificantes. Gracias a las técnicas de secuenciación masiva de nueva generación, durante la última década se reveló que decenas de miles de RNAs que no codifican para proteínas (llamados ncRNA, por RNAs no codificantes) conforman una clase de RNAs mucho más abundante y diversa en organismos vivos que la originalmente anticipada. Nuestro grupo se enfoca principalmente en el estudio de dos de estas clases de ncRNA, los microRNAs (miRNAs) y RNAs circulares (circRNAs).
RNA functions as a carrier of information from DNA to proteins through complex mechanisms. But instead of coding for proteins, certain RNA molecules regulate their expression or perform other non-coding functions. Thanks to next-generation sequencing techniques, during the last decade it has been revealed that tens of thousands of RNAs that do not code for proteins (called ncRNAs, for non-coding RNAs) make up a much more abundant and diverse class of RNAs in living organisms. than originally anticipated. Our group focuses mainly on the study of two of these classes of ncRNAs, microRNAs (miRNAs) and circular RNAs (circRNAs).
Líneas de Investigación / Research lines:
Los miRNAs son RNAs pequeños no codificantes que regulan una gran diversidad de procesos biológicos. Su función canónica es la de silenciar la traducción e inducir la degradación de RNAs mensajeros (mRNAs) a través de guiar a proteínas Argonauta a regiones 3′ no codificantes de los mRNA blancos. Algunos mRNA con inusualmente alta afinidad por miRNAs específicos revierten esta lógica, llevando a la degradación de los propios miRNAs (mecanismo conocido como TDMD por las siglas en inglés de Target RNA-Directed MiRNA Degradation). El TDMD puede ser inducido por virus llevando a la degradación de miRNAs específicos del huésped que favorecen la infección. Nuestro grupo descubrió que el TDMD es inusualmente potente en neuronas, aunque también es actuvo en otros tipos celulares. Aunque el TDMD es la principal via de degradación de los miRNAs inestables, los mecanismos que lo ejecutan y regulan se conocen solo parcialmente. Nuestro grupo busca dilucidar los mecanimos involucrados en dicho proceso. Aunque unos pocos casos se han descubierto recientemente, una pregunta abierta es cuáles son los RNAs blanco endógenos que disparan esta regulación en condiciones fisiológicas o patológicas. Nuestro grupo está explorando estas preguntas a través de aproximaciones bioinformáticas y experimentales con el fin de encontrar especies de RNA endógenas que tengan efecto en la estabilidad de miRNAs.
MiRNAs are small non-coding RNAs that regulate a wide variety of biological processes. Their canonical function is to silence translation and induce degradation of messenger RNAs (mRNAs) by guiding Argonaute proteins mostly to their 3′ untranslated regions of target mRNAs. Some mRNAs with unusually high affinity for specific miRNAs reverse this logic, leading to the degradation of the miRNAs themselves (a mechanism known as TDMD for Target RNA-Directed MiRNA Degradation). TDMD can be induced by viruses leading to the degradation of host-specific miRNAs that favor infection. Our group found that TDMD is unusually potent in neurons, but it is also active in other cell types. While TDMD is the main degradation pathway for unstable miRNAs, the mechanisms that execute and regulate it are only partially understood. Our group seeks to elucidate the mechanisms involved in this process. Although a few cases have been discovered recently, an open question is which are the endogenous target RNAs that trigger this regulation in physiological or pathological conditions. Our group is exploring these questions through bioinformatic and experimental approaches in order to find endogenous RNA species that have an effect on the stability of miRNAs.
Los circRNA son el producto de un modo particular de splicing alternativo que genera estructuras de RNA cerradas covalentemente, sin extremos 5′ y 3′ y por ende de una altísima estabilidad. Los circRNAs tienen pocas y diversas funciones conocidas, siendo la más estudiada la de “secuestrar” e inhibir a miRNAs específicos. Sin embargo, se desconoce si la topolgía circular de los circRNAs les confiere propiedades distintas de sus contrapartes lineales más allá de aumentar su estabilidad. Nuestro grupo busca entender si dicha “circularidad” les confiere propiedades bioquímicas y moleculares particulares, en particular en relación a su acción sobre la función y/o metabolismo de los miRNAs.
CircRNAs are the product of a particular alternative splicing mode that generates covalently closed RNA structures, free from 5′ and 3′ ends and therefore displaying a very high stability. CircRNAs have few and diverse known functions, the most studied being that of “sponging” and inhibiting specific miRNAs. However, it is unknown whether the circular topology of circRNAs confers properties different from their linear counterparts beyond increasing their stability. Our group seeks to understand if the “circularity” confers them particular biochemical and molecular properties, particularly in relation to their action on the function and/or metabolism of miRNAs.
Dada su excepcional estabilidad, es posible encontrar a los circRNAs en fuídos corporales como plasma y orina. Nuestro grupo forma parte de un equipo multidisciplinario junto con otros/as investigadores/as de nuestro instituto con el objetivo de explotar la inusual estabilidad y subsiguiente potencial de los circRNAs como biomarcadores en el cáncer de mama.
Given the exceptionally high stability, circRNAs can be found in body fluids such as plasma and urine. Given their exceptional stability, circRNAs can be found in body fluids such as plasma and urine. Our group is part of a multidisciplinary team with other researchers from our institute with the aim of exploiting the unusual stability and subsequent potential of circRNAs as biomarkers in breast cancer.
· Influence of circular RNA topology on microRNA stability. Federico Fuchs Wightman, Jerónimo Lukin, Sebastián Giusti, Laureano Bragado, Berta Pozzi, Paula González, Juan Pablo Fededa, Damian Refojo, Manuel de la Mata. BioRxiv preprint. doi: https://doi.org/10.1101/2022.04.11.487822.
· A DNA intercalating dye-based RT-qPCR alternative to diagnose SARS-CoV-2. Fuchs Wightman F, A Godoy Herz M, Muñoz JC, Stigliano JN, Bragado L, Nieto Moreno N, Palavecino M, Servi L, Cabrerizo G, Clemente J, Avaro M, Pontoriero A, Benedetti E, Baumeister E, Rudolf F, Remes Lenicov F, Garcia C, Buggiano V, Kornblihtt AR, Srebrow S, de la Mata M, Muñoz MJ, Schor IE, Petrillo E (2021). RNA Biology. DOI: https://doi.org/10.1080/15476286.2021.1926648
· Edición de genomas por CRISPR en investigación básica y aplicada. Palavecino M, de la Mata M. (2020). Revista Farmacéutica. 162(2): 9-35.
· Target RNAs Strike Back on MicroRNAs. Fuchs Wightman F, Giono LE, Fededa JP, de la Mata M. (2018). Front Genet. 9:435. doi: 10.3389/fgene.2018.00435.
· Turning the table on miRNAs. de la Mata M and Großhans H. (2018). Nat Struct Mol Biol. 25(3):195-197.
· Potent degradation of neuronal miRNAs induced by highly complementary targets. de la Mata M., Gaidatzis D., Vitanescu M., Stadler M.B., Wentzel C., Scheiffele P., Filipowicz W., Großhans H. (2015). EMBO Reports 16, 500-511.
· How Slow RNA Polymerase II Elongation Favors Alternative Exon Skipping. Dujardin G, Lafaille C, de la Mata M, Marasco LE, Muñoz MJ, Le Jossic-Corcos C, Corcos L, Kornblihtt AR. (2014). Mol Cell 54, 683-690.
· RNA polymerase II elongation at the crossroads of transcription and alternative splicing. de la Mata, M., Muñoz, M.J., Alló, M., Fededa, J. P., Schor, I.E., and Kornblihtt, A.R. (2011). Genetics Research International. doi:10.4061/2011/309865
· Chromatin and Alternative Splicing. Alló, M., Schor, I.E., Muñoz, M.J., de la Mata, M., Agirre, E., Valcarcel, J., Eyras, E., and Kornblihtt, A.R. (2011). Cold Spring Harb Symp Quant Biol. doi:10.1101/sqb.2010.75.023
· First come, first served revisited: factors affecting the same alternative splicing event have different effects on the relative rates of intron removal. de la Mata, M., Lafaille, C., and Kornblihtt, A.R. (2010). RNA 16, 904-912.
· The carboxy terminal domain of RNA polymerase II and alternative splicing. Muñoz, M.J., de la Mata, M., and Kornblihtt, A.R. (2010). Trends Biochem Sci 35, 497-504.
· Control of alternative splicing through siRNA-mediated transcriptional gene silencing. Alló, M., Buggiano, V., Fededa, J.P., Petrillo, E., Schor, I., de la Mata, M., Agirre, E., Plass, M., Eyras, E., Elela, S.A., Klinck, R., Chabot, B. and Kornblihtt, A.R. (2009). Nat Struct Mol Biol 16, 717-724.
· DNA damage regulates alternative splicing through inhibition of RNA polymerase II elongation. Muñoz, M.J., Perez Santangelo, M.S., Paronetto, M.P., de la Mata, M., Pelisch, F., Boireau, S., Glover-Cutter, K., Ben-Dov, C., Blaustein, M., Lozano, J.J., Bird, G., Bentley, D., Bertrand, E. and Kornblihtt, A.R. (2009). Cell 137, 708-720.
· SF2/ASF regulates proteomic diversity by affecting the balance between translation initiation mechanisms. Blaustein, M., Quadrana, L., Risso, G., de la Mata, M., Pelisch, F., and Srebrow, A. (2009). J Cell Biochem 107, 826-833.
· The transcriptional cycle of HIV-1 in real-time and live cells. Boireau, S., Maiuri, P., Basyuk, E., de la Mata, M., Knezevich, A., Pradet-Balade, B., Backer, V., Kornblihtt, A., Marcello, A., and Bertrand, E. (2007). J Cell Biol 179, 291-304.
· RNA polymerase II C-terminal domain mediates regulation of alternative splicing by SRp20. de la Mata, M., and Kornblihtt, A.R. (2006). Nat Struct Mol Biol 13, 973-980. Comment in: Lynch K.W. (2006) Cotranscriptional splicing regulation: it’s not just about speed. Nat Struct Mol Biol. 13(11):952-3.
· Multiple links between transcription and splicing. Kornblihtt, A. R., de la Mata, M., Fededa, J. P., Muñoz, M. J. & Nogués, G (2004). RNA 10:1489–1498.
· A slow RNA polymerase II affects alternative splicing in vivo. de la Mata, M., Alonso, C.R., Kadener, S., Fededa, J.P., Blaustein, M., Pelisch, F., Cramer, P., Bentley, D., and Kornblihtt, A.R. (2003). Mol Cell 12, 525-532. Comment in: Proudfoot NJ (2003) Dawdling polymerases allow introns time to splice. Nat Structural Biology. 10(11): 876-878.
· An early ancestor in the evolution of splicing: a Trypanosoma cruzi serine-arginine-rich protein (TcSR) is functional in cis-splicing. Portal, D., Espinosa, J.M., Lobo, G.S., Kadener, S., Pereira, C.A., de la Mata, M., Tang, Z., Lin, R.J., Kornblihtt, A.R., Baralle, F.E., et al. (2003). Mol Biochem Parasitol 127, 37-46.
· Control of alternative pre-mRNA splicing by RNA Pol II elongation: faster is not always better. Nogués, G., Kadener, S., Cramer, P., de la Mata, M., Fededa, J.P., Blaustein, M., Srebrow, A., and Kornblihtt, A.R. (2003). IUBMB Life 55, 235-241.
· Antagonistic effects of T-Ag and VP16 reveal a role for RNA pol II elongation on alternative splicing. Kadener, S., Cramer, P., Nogues, G., Cazalla, D., de la Mata, M., Fededa, J.P., Werbajh, S.E., Srebrow, A., and Kornblihtt, A.R. (2001). EMBO J 20, 5759-5768.
· Coordination between transcription and pre-mRNA processing. Cramer, P., Srebrow, A., Kadener, S., Werbajh, S., de la Mata, M., Melen, G., Nogues, G., and Kornblihtt, A.R. (2001). FEBS Lett 498, 179-182.
· Identification of a CD8alpha(+) dendritic cell subpopulation in rat spleen and evaluation of its OX-62 expression. de la Mata, M., Riera, C.M., and Iribarren, P. (2001). Clin Immunol 101, 371-378.
· [Induction of primary immune response by rat dendritic cells]. de la Mata, M., Correa S.G., Riera, C.M., and Iribarren, P. (2000). Rev Fac Cien Med Univ Nac Cordoba 57(2), 219-225.under
Papers
-
noticias de la mata
28/10/2019 Noticias / Medios - Mata
Integrantes del grupo
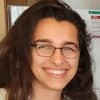
Faustina Salgado
Estudiante
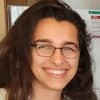
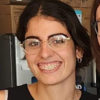
Maribel Gándara
Estudiante
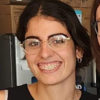
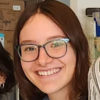
Lic. María Luz Pierelli
Becaria doctoral
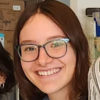
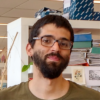
Dr. Marcos Palavecino
Becario postdoctoral
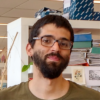
Miembros Anteriores
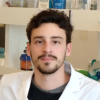
2